Better Microscope for Studying Proteins in Living Cells
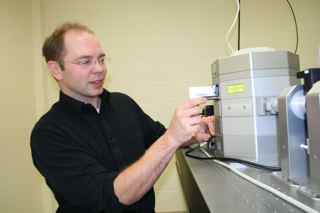
Inventor: Adam Hoppe
Article and Photo by Lance Nixon
A scientist who grew up tinkering on backyard inventions with his father and grandfather on a Minnesota farm has moved on to bigger challenges: building a better microscope for studying proteins in living cells.
A major grant from the National Science Foundation is funding the South Dakota State University researcher’s work building a high-tech microscope that will allow scientists to make movies of unprecedented resolution to show biochemical machinery at work inside living cells.
Assistant professor Adam Hoppe in SDSU’s Department of Chemistry and Biochemistry has won an NSF CAREER award, awarded to new or young investigators through a competitive application process. Researchers are allowed three attempts to get one of these grants. Hoppe, who grew up on a farm near Crookston, Minn., landed it on his first try for his ongoing efforts to develop new instrumentation to study protein-to-protein interactions inside the living cell.
Hoppe’s $785,000 grant not only does cutting-edge science, it also grooms the next generation of scientists. In addition to building the new instrument according to his own design — actually refining and enlarging the instrument he’s already designed — Hoppe will carry out a three-part educational plan. That includes training graduate students and also bringing high school teachers in to get real-life laboratory experience that can help them to the classroom. The third part of that educational component is to use the Internet to disseminate information about the research and explain to a lay audience why it’s important.
Hoppe, a biophysicist, is part of the SDSU-based 2010 Center for Biological Control and Analysis by Applied Photonics, or BCAAP. The center is made up of researchers who use light as one of the tools either to control biochemical processes, or — in the case of Hoppe’s project — to analyze biochemical processes.
“What really motivates the development of this microscopy tool for me is, if you want to understand cellular biochemistry, you’ve got to study it in the cell,” Hoppe said. “You can take a car apart and look at each piece individually, that’s what classical biochemistry has done, and we’ve learned a tremendous amount by doing that. But if you want to know how the car runs, you have to understand how the pieces are put together, how they’re organized, and how the organization works together. That’s what I think this kind of technology is going to do. It is going to let us watch biochemical machinery operate in its environment.”
The microscope will shed light on something strikingly simple yet important regarding molecular pathways, or the interactions among molecules in a cell that lead to a certain result or accomplish a specific cell function.
“When biologists draw pictures of how a molecular pathway works inside of a cell, they’re usually drawing arrows between multiple proteins. Those arrows usually represent some kind of biochemical event,” Hoppe said. “What you find is that the majority of them actually represent something very, very simple, something very fundamental, and it is simply one protein touching another protein. You can have two proteins that are sitting there doing nothing in the cell. However, if they touch each other, then one or both of them can become active, that is, do something biochemically significant in the cell. It’s a theme that reoccurs in biology, that proteins are regulated by their contact with other proteins.”
The microscope work will build on Hoppe’s experience designing instruments that use an optical phenomenon called fluorescence resonance energy transfer, or FRET. That phenomenon depends on special molecules called fluorophores that absorb energy and give off energy at a different wavelength, causing the molecule to be fluorescent.
That phenomenon allows those special molecules of two different colors — say, a blue one and a yellow one — to exchange energy. But that exchange process happens only over very short distances — about 5 nanometers, or a billionth of a meter.
“What was very clear to me and some others in the field was that you should be able to take this phenomenon and extend it to high-resolution imaging to map when and where the protein interactions are inside the cell,” Hoppe said.
Professor Joel Swanson, who supervised Hoppe’s Ph.D. work at the University of Michigan and later kept him on as a postdoctoral researcher and then as a faculty researcher, said Hoppe made a key contribution by realizing that scientists were discarding a potential source of information.
“He is an extraordinary scientist. The methods he invented when he was in Michigan transformed our research program. Prior to his work, labs could see where protein-protein interactions were happening inside cells, but they could not measure the magnitudes of those interactions,” Swanson said. “Adam's chief initial insight was that the fluorescence others were removing as background noise contained information about the number of interacting molecules inside the cell. With that realization, he devised mathematical processing methods for calibrating microscopes and quantifying the chemistries inside the cells.”
Eventually, Hoppe said, the instrument could be applied to very specific problems to try to answer specific questions that may directly impact human health. Hoppe has already used the technology to study factors regulating the process of cell eating, or phagocytosis, an essential activity of the immune system. At SDSU, Hoppe hopes to use the technology to probe deeper into the biochemistry of the immune system and to assist colleagues who are studying the way influenza viruses assemble, for example.